Imagine a playful game with friends, where everyone sits on the lap of the person behind them, forming a perfect circle. The weight is evenly shared, with no one carrying the load alone and no one relying on outside support. This is exactly how a reciprocal frame (RF) structure works, where each beam leans on the next to create a self-supporting loop that needs no central pillar. Like the lap circle, RF structures balance weight effortlessly.
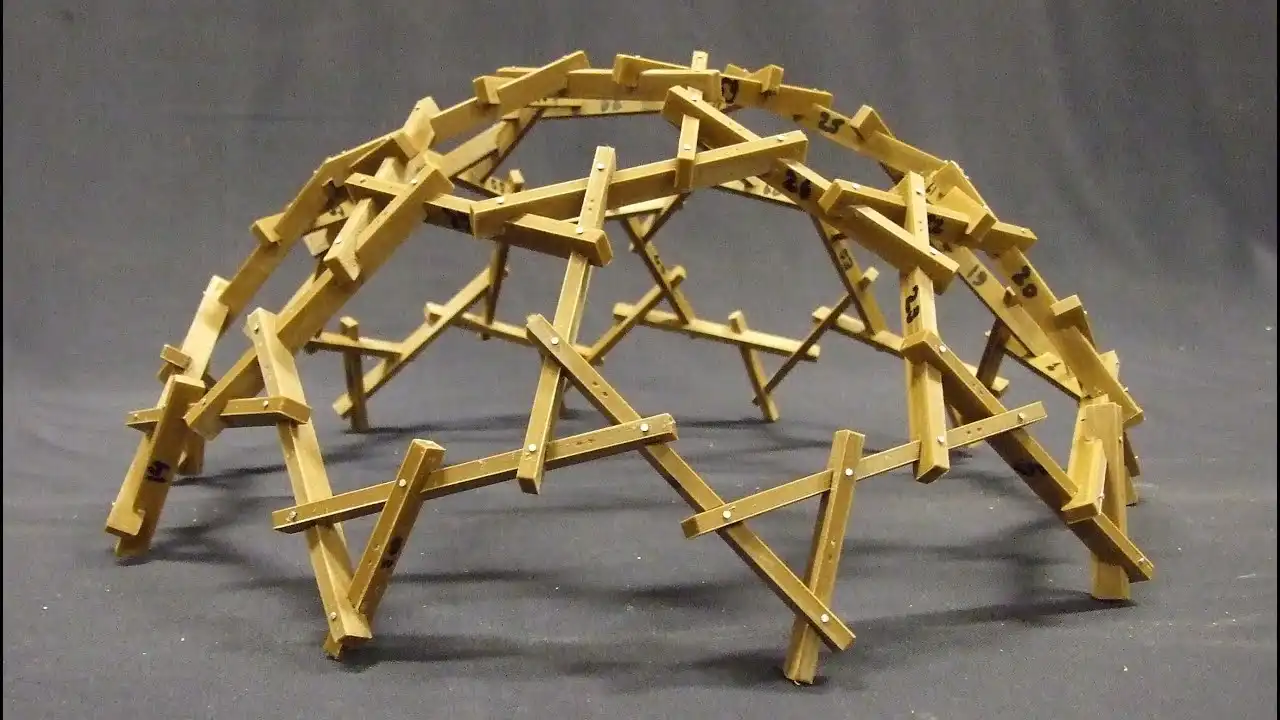
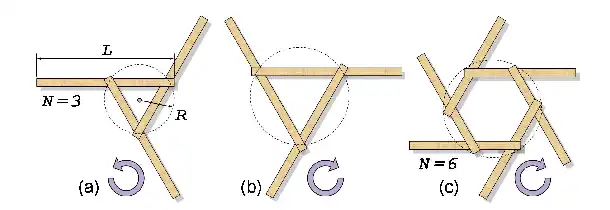
Typically, the beams are arranged in a radial or polygonal grid, with carefully calculated angles and lengths to ensure they interlock securely. This tight interlocking allows for efficient load distribution across the structure while also providing enough flexibility to handle dynamic forces, such as wind or minor ground shifts. This makes RF structures resilient, versatile, and suitable for various architectural applications. In this article, we’ll showcase five modern examples using this clever technique in fascinating ways. But first, let’s understand the historical overview of Reciprocal Frame Structures.
Historical Overview of RF Structures
Some scholars date back to the origin of RF structures to early human civilizations, such as neolithic huts, nomadic yurts, and Navajo hogans. These primitive homes did not exactly employ RF structure techniques but rather similar methods of weaving various materials together to create roofs.
The actual emergence of said structures was in 12th century Japan, where Buddhist monk Chogen (1121 – 1206) developed a spiral layering of wood technique for the construction of temples and shrines. The Great South Gate of Todai-ji in Nara and Jodo Hall in Hyogo Prefecture are some of the earliest iconic examples of reciprocal frame structures.
At that time, German architects named these structures “Mandala Roofs” because their geometric forms reminded them of Buddhist mandalas. A century later, medieval artist Villard de Honnecour (1200 -1250) came up with a clever solution for spanning large spaces by arranging four beams in a reciprocal frame (RF) configuration. This early use of reciprocal framing was quite innovative.
Fast forward to the Gothic era, and we start seeing elements of these reciprocal frames in timber roof trusses for cathedrals. Then, Leonardo da Vinci sketched a similar beam arrangement in his Codex Madrid during the Renaissance. His repeated use of this reciprocal frame concept for supporting bridges shows how influential and versatile it became in structural design.
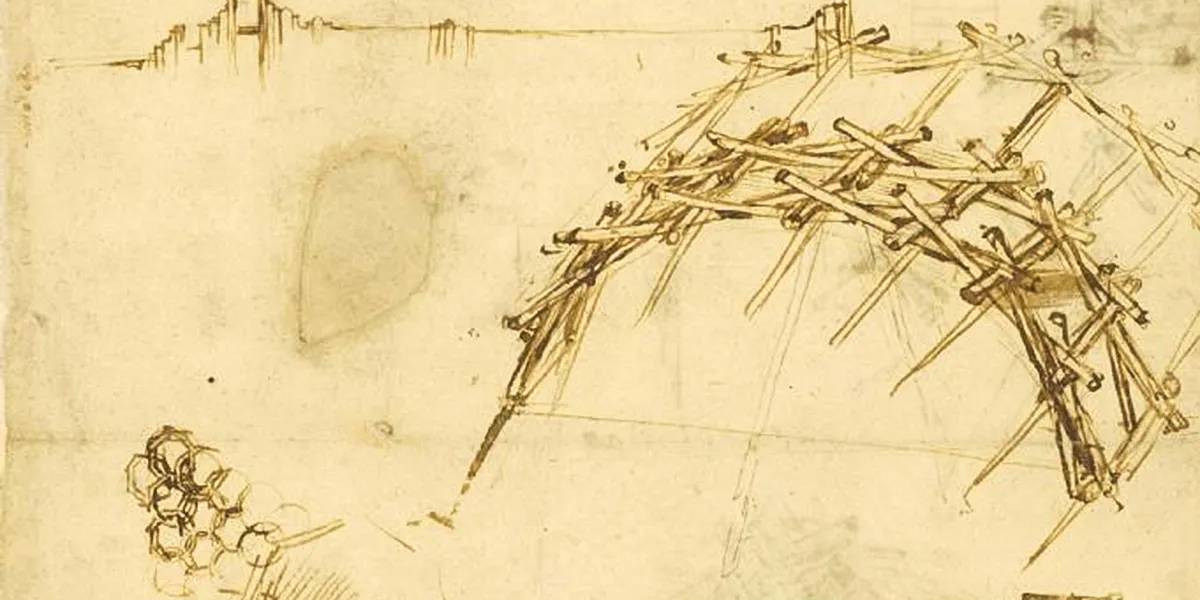
The technique was rediscovered and formalized by architect and engineer Graham Brown in the 1980s, who patented the term “reciprocal frames” inspired by the way beams mutually support each other. His work, particularly in the UK, pioneered the modern use of RF structures and showcased how simple timber or even steel beams could create complex yet geometrically precise designs.
In the modern era, architects began to explore RFs not just for traditional wood-based designs but as an adaptable method for various materials. The digital age and the rise of parametricism, a design approach that uses algorithms to generate complex forms, further revolutionized RF applications. Architects like Zaha Hadid and Patrik Schumacher integrated RF concepts into their parametric designs. This opened a whole new way of creating fluid forms that push the boundaries of architectural expression.
Let’s explore some of the best modern examples of Reciprocal Frame Structures:
Serpentine Pavilion
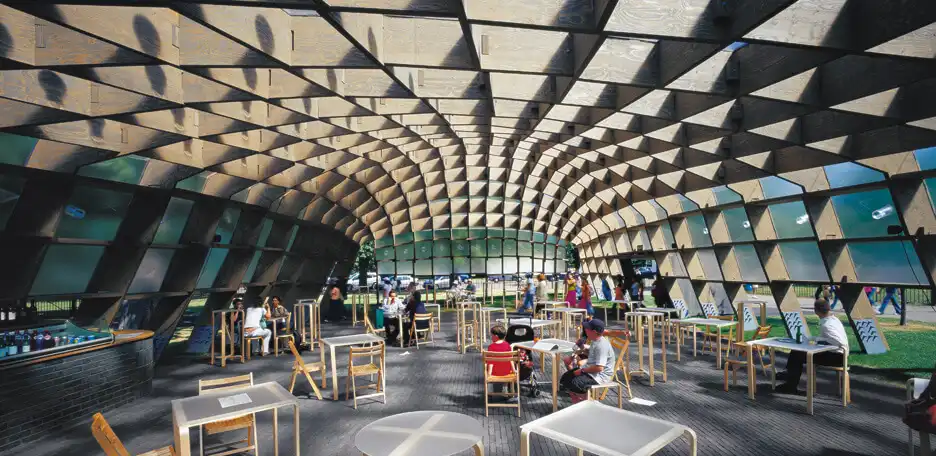
Architect: Álvaro Siza, Eduardo Souto de Moura, and Cecil Balmond
Year: 2005
The 2005 Serpentine Gallery Pavilion, designed by Álvaro Siza, Eduardo Souto de Moura, and Cecil Balmond, featured a striking 400-meter square arching grid of laminated spruce. They innovated the use of reciprocal structures by arranging interlocking elements of two grid-unit lengths in a mutually supporting pattern. This way, each element had mortice-and-tenon connections while maintaining the overall grillage bending stiffness.
The pavilion’s translucent clad panels with solar-powered lights gently nod to the lamella barrel-vaulted roofs of 1920s Germany. The modern twist was to vary each timber element in length and angle. The pavilion’s design intention sought to establish a dialogue between the old neoclassical house and the new structure, which resulted in a curvaceous form that complements the park’s landscape.
Reciprocal Frame Installation
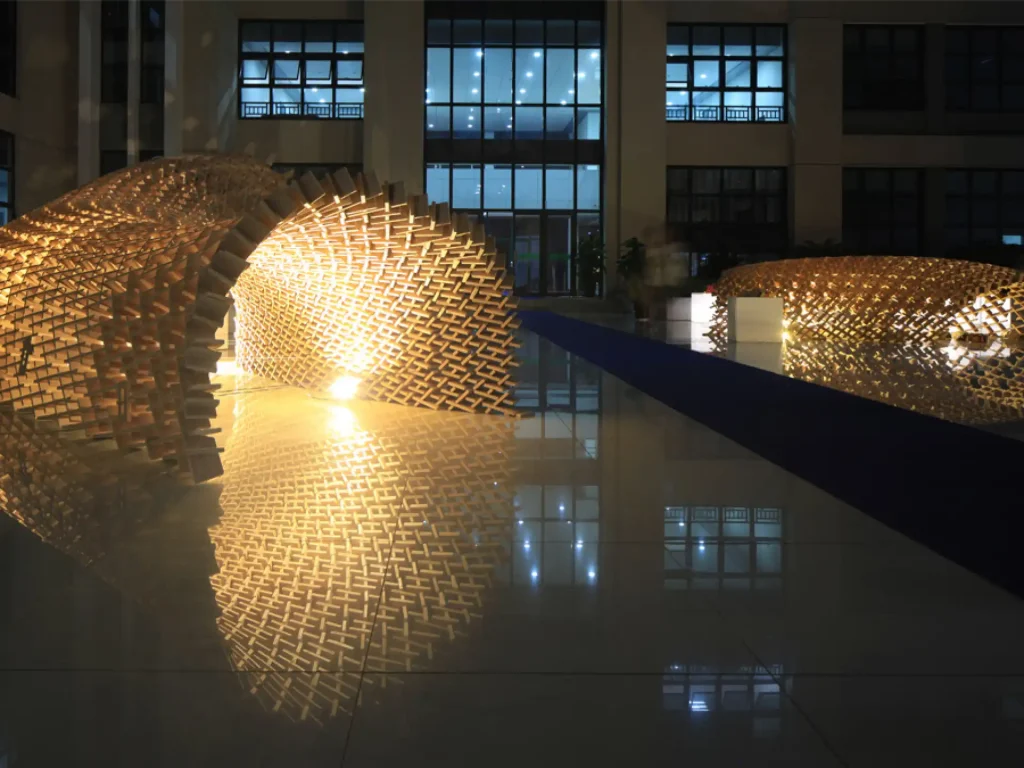
Architect: Nathan Melenbrink, Samo Pedersen and Shibu Raman
Year: 2014
At Nottingham’s Ningbo University Campus, students Nathan Melenbrink, Samo Pedersen, and Shibu Raman designed a pavilion that warps up their four years of academic work. Created through collaborative weekend workshops, the pavilion features a three-part, parametrically-designed structure assembled in the architecture school’s atrium.
Despite their initial inexperience with digital and parametric design, they engaged in extensive trials and mockups to end up with a final structure that demonstrates precision and fluidity in plywood. The pavilion, named Reciprocal Frame, consists of 3,019 notched plywood segments. To give a more powerful effect, interior lights highlight the pavilion’s intricate mesh surface, creating a dynamic play of light and shadow. This design encourages visitors to engage with the space in unique and unexpected ways, enriching their overall experience of the exhibition.
Shanghai Bamboo Pavilion
Architect: Keio University and Tongji University
Year: 2018
The Shanghai Bamboo Pavilion, a collaborative effort between Ban Laboratory at Keio University and Tongji University in China, represents an innovative exploration of bamboo in architectural design. To capture the structure’s elegance and complexity, the team employed a computational model that meticulously analyzed the behavior of the reciprocal frame system. One of the key challenges was the use of bamboo with rectangular cross-sections, which complicated the goal of achieving seamless tangency between frame elements.
The shallow dome design and the frame’s thickness and preset engagement lengths introduced significant internal strain and deformation. The team developed two unique models to overcome these constraints: a circle-tangent model and a custom bend-conform model. These models employed gradient-descent optimization to ensure flawless engagement. The result was a pavilion that blends craftsmanship with computational precision and demonstrates bamboo’s architectural potential.
Future Tree
Architect: Gramazio Kohler Research Group
Year: 2019
Designed by Gramazio Kohler, the Future Tree is an innovative project at the Basler & Hofmann office extension in Esslingen, Switzerland. The top part of the Future Tree is a canopy made of 380 timber pieces arranged in a reciprocal frame pattern. This structure is held up by a concrete column, with two sides attached to the building, while the other corner extends outward without direct support (cantilevering).
The frame’s geometry, optimized for structural performance, adjusts stiffness through reciprocal knot openings. The timber, made from acetylated pine, is assembled with screws and tension cables, a great utilization of industrial robotic fabrication. The concrete column, constructed using the “Eggshell” process, employs a robotically 3D-printed, ultra-thin formwork with fast-hardening concrete. This technique minimizes waste and allows for structurally efficient forms. This also introduces a sustainable approach to modern concrete construction.
Reciprocal Timber Shell
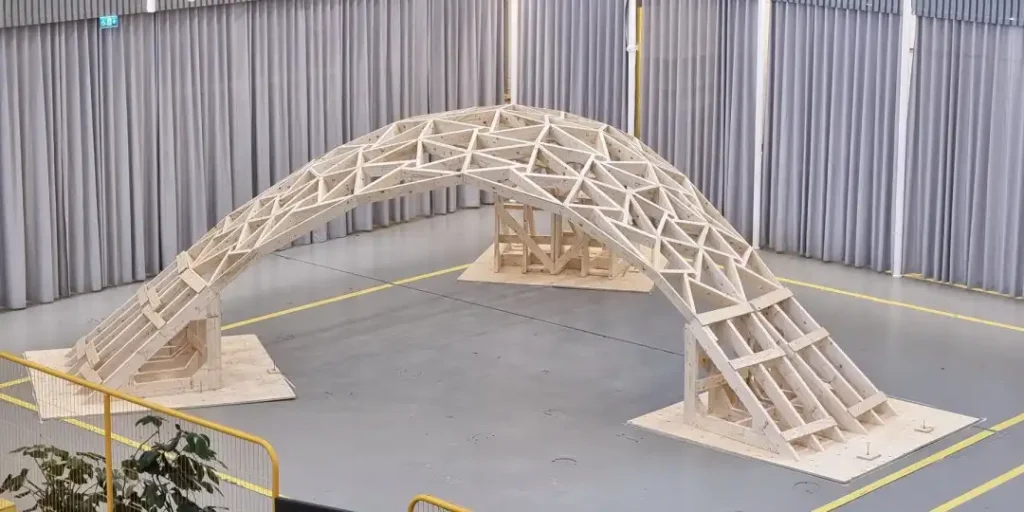
Architect: Chalmers University of Technology and Augsburg University of Applied Sciences
Year: 2024
The Reciprocal Shell, a collaboration between Chalmers University of Technology and Augsburg University of Applied Sciences, redefines the potential of timber in architecture. Designed to overcome the geometric challenges of free-form shell structures, this hybrid system integrates cross-bracing and reciprocal nodes to create a strong and stable framework.
A case study featuring a 7.5-meter demonstrator showcased the efficiency of its design, which simplifies construction by using 144 prefabricated wood elements. Utilizing multi-axis robotic cutting, the system achieves remarkable precision, allowing rapid assembly and minimal waste. This sustainable approach, driven by advanced CAD software, highlights the potential for transforming timber construction into an eco-friendly practice for the future.
Leave a comment